In a recent article in Nature Communications, researchers from DTU Physics and DTU Mechanical Engineering report on a novel route towards micromechanical resonators that sustain their motion for so long that they may enable fundamental tests of quantum mechanics at room temperature.
In the project, the researchers sought out to implement topology optimization to generate new ultra-high quality membrane resonator designs, and experimentally verify their performance. And it paid off. Compared to earlier results, the new optimized designs resulting from the researchers’ efforts show a greatly enhanced performance, enabling 4 quantum coherent oscillations at room temperature. For traditional resonator designs of the same size and simplicity this is completely unmatched.
“Topology optimization has highlighted a new design optimization strategy to further isolate a resonator’s mechanical vibrations, paving the way for ultra-coherent mechanical resonators”, says Dennis Høj, who recently finished his PhD at DTU Physics and has been the driving force behind the work.
Ultra-coherent mechanical resonators are devices with ultra-low mechanical losses, leading to exceptionally long-lived vibrations. This property makes them an interesting platform for a range of exotic applications, including high-precision sensing, memories in quantum computers, and testbeds for fundamental optomechanical tests of quantum mechanics.
“In bigQ, we strive to expose quantum mechanical behavior at large scales. This work has brought us an important step closer, and I am sure the results will impact the field of quantum optomechanics in general. Our resonators are of course still micrometer-sized objects, but they are visible to the naked eye and contain billions of atoms - very macroscopic compared to single quanta”, says Prof. Ulrik Lund Andersen, leader of the bigQ research center at DTU Physics.
An alternative approach is needed
Traditionally, low-loss mechanical resonators are fabricated by stressing the material to just below the breaking point. As an analogy, one can think of a violin string: By increasing the string tension the frequency is increased. This in turn increases the energy of the vibrations without increasing the loss, thereby increasing the overall quality factor which is a measure of the resonator’s energy loss rate. However, at some point you reach the material’s breaking point, and the tension cannot be increased any further.
It turns out the design of a membrane resonator under tension leaves a lot of room for improving the quality. Trampoline shaped designs have already been investigated as well as using mechanical crystal structures (phononic crystals) to shield the mechanical resonators. However, these are based on human intuition and might leave out a plethora of high-performing, but hard to imagine, designs.
This is where the concept of topology optimization comes in. It is a computational tool that works by iteratively adding or removing material in a design domain until it converges towards a new and fully optimized design. Topology optimization has previously demonstrated great ability to generate remarkable designs, including optimization of a full-scale airplane wing, and the girder of a suspension bridge.
“Our group has vast experience in applying topology optimization to a broad range of applications, both at large and small scales, but never before with the purpose of pushing mechanical resonators into the quantum regime. This has been an exciting project for us”, says Prof. Ole Sigmund from DTU Mechanical Engineering.
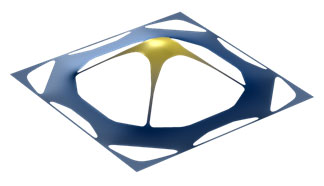
An example of a non-intuitve design that has emerged out of the topology optimization algorithm. It clearly shows features along the edges that deviate from the ordinary design, and which adds significantly to the oscillation lifetime of the central four-legged trampoline.
The power of synergy
The work is a prime example of the synergies that can be activated by bridging different research fields – in this case a unique mix of basic theoretical developments, advanced numerical procedures, inverse design, nano-fabrication and experiments. Spurred by the extreme requirements for quantum physics experiments this has led to new insights into the development of ultra-coherent resonators and highlighted the untapped potential of topology optimization in the field of optomechanics.
With a tried and tested implementation in place, next step is to build on the experience gathered and apply it to other types of mechanical resonators. The ambition is to continue the collaboration and push the limits of ultra-coherent mechanical resonators even further. Hopefully, this will open up new avenues within quantum sensing and optomechanics.
The work has been carried in a collaboration between the DNRF Center of Excellence bigQ at DTU Physics and the Villum Investigator Project InnoTop of the TopOpt group at DTU Mechanical Engineering.
The results were published in Nature Communications on October. 1, 2021